Summary
Confocal microscopy offers superior resolution compared to conventional microscopy by eliminating out-of-focus light, providing optical sectioning, reducing background noise, and employing optimized optical designs. In this article, we first tell what out-of-focus fluorescence is, and how to mitigate the out-of-focus fluorescence. Then, two important concepts are introduced, which explain why resolution and contrast can affect image quality. Third, we introduce how confocal microscopy block the out-of-focus fluorescence, and how the confocal microscopy improves the image resolution and contrast. Last, how to create 2D images using the confocal microscopy by single point scanning, line scanning, and spinning disk.
Out-of-focus fluorescence
The problem of out-of-focus fluorescence in microscopy is commonly referred to as background fluorescence or out-of-focus light. It occurs when the emitted fluorescence light from the sample interacts with other elements in the imaging system, such as the surrounding medium, the microscope's optics, or the detector, resulting in a blurred or hazy background signal.
Several factors contribute to the presence of out-of-focus fluorescence:
Light scattering: When the excitation light passes through the sample, it can scatter off the surrounding medium or other structures within the sample. Scattered light can then contribute to the background signal, especially if it is collected by the detector.
Refractive index mismatches: In some samples, there can be a refractive index mismatch between the sample and the surrounding medium or mounting medium. This mismatch can lead to the bending or scattering of light rays, causing them to deviate from the desired imaging path and contribute to the out-of-focus signal.
Optical aberrations: Imperfections in the microscope's optical components, such as lenses or mirrors, can cause optical aberrations. These aberrations can result in the blurring and spreading of the fluorescence signal beyond the focal plane, leading to out-of-focus fluorescence.
Detector sensitivity: Detectors used in fluorescence microscopy have a certain degree of sensitivity to light, including fluorescence emitted from out-of-focus regions. Even if the sample is properly illuminated and focused, the detector may still pick up some background fluorescence, leading to a reduction in image contrast.
Autofluorescence: Some samples exhibit inherent autofluorescence, where certain molecules or structures naturally emit fluorescence when excited by light. Autofluorescence can contribute to the overall background signal, making it challenging to differentiate it from the specific fluorescence of interest.
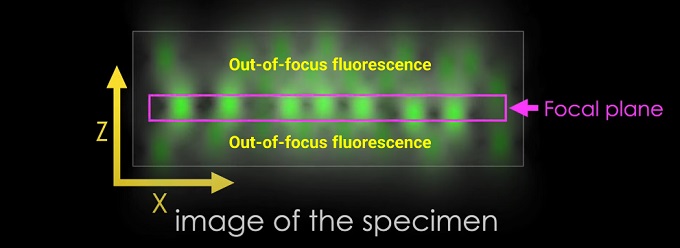
Out-of-focus fluorescence
How to mitigate the out-of-focus fluorescence?
To mitigate the problem of out-of-focus fluorescence, several techniques can be employed:
Proper sample preparation: Using appropriate mounting media, clearing agents, or refractive index matching solutions can help minimize refractive index mismatches and reduce light scattering within the sample.
Optical sectioning techniques: Employing imaging techniques that provide optical sectioning capabilities, such as confocal microscopy or two-photon microscopy, can selectively capture fluorescence from the focal plane while rejecting out-of-focus light. These techniques help improve image contrast and resolution.
Deconvolution algorithms: Post-acquisition image processing techniques, such as deconvolution algorithms, can be applied to remove the contribution of out-of-focus fluorescence. Deconvolution algorithms estimate and correct for the blurring effects caused by optical aberrations, resulting in sharper and more focused images.
Spectral separation: Utilizing fluorescence probes with distinct emission spectra and employing spectral imaging or spectral unmixing techniques can separate the specific fluorescence signal from the background or autofluorescence components.
Optimization of imaging parameters: Adjusting imaging parameters like illumination intensity, exposure time, and detector settings can help optimize the signal-to-background ratio and minimize the impact of out-of-focus fluorescence.
By employing these strategies, researchers can mitigate the effects of out-of-focus fluorescence and improve the quality of fluorescence microscopy images.
Resolution and contrast in microscopy
Resolution and contrast are two essential parameters in microscopy that determine the quality and interpretability of the images obtained.
Resolution: Resolution refers to the ability of a microscope to distinguish two closely spaced objects as separate entities. It determines the level of detail and sharpness in an image. In other words, it quantifies the smallest distance between two points in a sample that can be resolved by the microscope.
The resolution of a microscope is influenced by several factors, including the numerical aperture (NA) of the objective lens, the wavelength of light used for imaging, and the quality of the optics. The Abbe diffraction limit, formulated by Ernst Abbe, provides a theoretical description of the maximum achievable resolution in a conventional light microscope. According to this limit, the resolution is approximately half the wavelength of light divided by the NA.
Different microscopy techniques have been developed to overcome the diffraction limit and achieve higher resolution. Examples include confocal microscopy, structured illumination microscopy (SIM), stimulated emission depletion (STED) microscopy, and super-resolution techniques like stochastic optical reconstruction microscopy (STORM) and photoactivated localization microscopy (PALM). These methods employ various principles to enhance resolution and reveal finer details in the sample.
Contrast: Contrast refers to the distinguishability or visual separation of different features or objects within an image. It determines the ability to discriminate between regions of interest and the background or between different components within the sample.
Contrast in microscopy can be influenced by factors such as the intrinsic properties of the sample, the staining or labeling techniques employed, and the imaging parameters. Different microscopy techniques may have inherent contrast mechanisms, while others may require additional sample preparation steps to enhance contrast.
Some common methods to enhance contrast in microscopy include:
Staining: The application of specific dyes or fluorophores to selectively label or highlight particular structures or components of interest within the sample.
Phase contrast microscopy: A technique that exploits the phase shifts caused by transparent specimens to generate contrast in the resulting image.
Differential interference contrast (DIC) microscopy: Also known as Nomarski interference contrast or DIC microscopy, this technique uses polarized light and optical prisms to create contrast by exploiting differences in refractive index and thickness in the sample.
Darkfield microscopy: In this technique, the specimen is illuminated with oblique or grazing incidence light, and only the scattered light is collected by the objective lens. This creates a dark background with bright, scattered light from the sample, enhancing contrast.
Fluorescence microscopy: Fluorescent labels or markers can be used to selectively label specific components within the sample, allowing for high contrast imaging of those components against the background.
Differential staining: Using different dyes or stains that preferentially bind to different components of the sample, leading to differential staining and contrast enhancement.
By optimizing imaging parameters, employing appropriate contrast-enhancing techniques, and selecting the most suitable microscopy method for the specific application, researchers can improve both resolution and contrast, leading to clearer and more informative microscopy images.
Confocal principle
The principle of confocal microscopy is based on the concept of optical sectioning, which enables the acquisition of high-resolution, three-dimensional images of biological specimens. Unlike traditional wide-field microscopy, where the entire specimen is illuminated and detected simultaneously, confocal microscopy selectively illuminates and detects a single focal plane at a time.
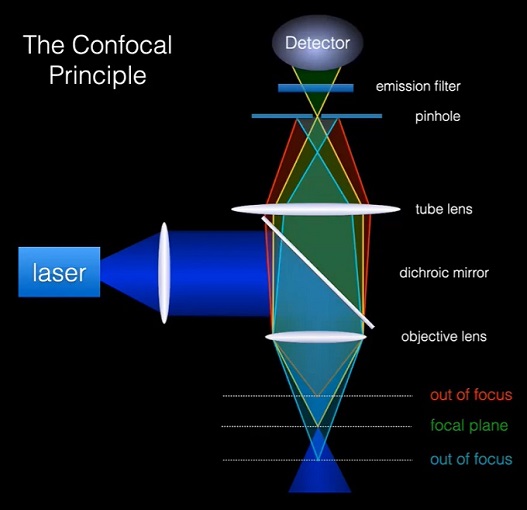
Principle of confocal microscopy
The key components and principles of confocal microscopy are as follows:
Point illumination: In confocal microscopy, a focused laser beam is used to illuminate a small point within the specimen. This laser beam is typically focused through the objective lens, and its diameter is adjusted to achieve optimal resolution.
Pinhole aperture: After passing through the specimen, the emitted fluorescence or reflected light is collected by the same objective lens. However, before reaching the detector, the collected light passes through a pinhole aperture. The pinhole blocks the light emitted or reflected from out-of-focus regions of the specimen, allowing only the light originating from the focal plane to pass through.
Scanning mechanism: To generate an image, the illuminated spot is scanned across the specimen in a raster pattern. This scanning can be achieved using various methods, such as galvanometer mirrors, acousto-optic deflectors, or spinning disks with spiral patterns. By scanning the laser beam point-by-point, a complete two-dimensional image of the focal plane is generated.
Confocal detection: The light passing through the pinhole is directed to a detector, such as a photomultiplier tube (PMT), which converts the light signal into an electrical signal. The detector's output is then used to construct the image.
The benefits and working principles of confocal microscopy are as follows:
Optical sectioning: By selectively illuminating a single focal plane and blocking out-of-focus light, confocal microscopy provides excellent optical sectioning capabilities. This enables the acquisition of sharp and well-defined images of thin slices or focal planes within thick specimens.
Improved resolution: The elimination of out-of-focus light and rejection of scattered light leads to improved axial and lateral resolution compared to wide-field microscopy. The pinhole aperture restricts the detection of light to a confined region, resulting in better image contrast and resolution.
3D imaging: By sequentially scanning multiple focal planes at different depths within the specimen, confocal microscopy allows the reconstruction of three-dimensional image stacks. These stacks can be visualized and analyzed to observe the spatial distribution of structures or processes within the specimen.
Reduction of background noise: Confocal microscopy's ability to reject out-of-focus light and minimize background noise improves image clarity and contrast. This is particularly useful when imaging samples with high levels of autofluorescence or when differentiating specific fluorescent signals from background fluorescence.
Confocal microscopy finds applications in various fields, including cell biology, neurobiology, developmental biology, and biomedical research. Its optical sectioning capability and improved resolution make it a valuable tool for visualizing and studying complex biological structures and processes within specimens.