Time-resolved photoluminescence (TR-PL) spectroscopy is a fundamental and versatile technique to probe carrier dynamics and their relaxation pathways in various photo-sensitive chemicals, physical and biological systems.
There are several techniques for studying photoluminescence lifetime. Among them, Time-Correlated Single Photon Counting (TCSPC) is the most common technique for fluorescence lifetime measurements. It allows to measures fluorescence decay time from 10sps to a few hundred nanoseconds producing a wealth of information of the sample. This application note will discuss the basics of luminescence, its application in the spectroscopy and microscopy, TCSPC setup and necessary components in detail. | 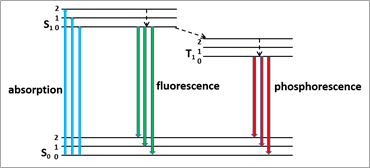
|
SIMTRUM Developed TCSPC System for SPAD , APD Testing Solution,
for details working principle and setup please click here
Setup-Guide: Luminescence and Time Correlated Single Photon Counting
+Time Correlated Single Photon Counting +Ultrafast optics +Avalanche Photodiode +Single Photon Counting Module +Time controller +Nanodiode
Contents
5.2 Equipment List
Application of photoluminescence
Fluorescence is a radiative relaxation process arising from charge relaxation from excited to ground state, also known as radiative recombination or radiative quenching.
Measurements of the absolute photoluminescence intensity at steady-state and its time dependence at ultrashort time scales will be used to assess the competing radiative and non-radiative e-h recombination pathways (e.g. charge transfer, energy transfer, Auger recombination, surface traps and defects etc.). In particular, time-resolved photoluminescence (TRPL) is a powerful spectroscopy technique to probe carrier dynamics and their relaxation pathways in semiconductor nanostructures. Through monitoring the PL decay dynamics of emitters, information on the radiative and non-radiative recombination rates could be extracted. In general, we can obtain this information from analysing decay lifetime of fluorescence.
-Charge transfer
-Energy transfer
-Formation of trap states
-Quenching of electron-hole
-Dynamic of excited-states
For example, the formation of charge-transfer states in the donor-acceptor photovoltaic hinders radiative recombination and to quench photoluminescence significantly. Hence, photoluminescence measurements provide evidence of charge transfer through the yield and the rate of radiative emission. In conjunction with transient absorption photoluminescence decay data can provide complementary information about excited-states.
Fluorescence lifetime imaging spectroscopy is another application of TRPL in biology. It can be used as an imaging technique in confocal microscopy, two-photon excitation microscopy, and multiphoton tomography.
Time Correlated Single Photon Counting (TCSPC) Technique
When a sample (usually a dye) is excited, it decays to the ground state in time, emitting a photon in the process. Since fluorescence lifetimes are typically in the range of several picoseconds to few nanoseconds, we need an accurate and sensitive technique to measure lifetime. Transient absorption spectroscopy is not the right choice to measure fluorescence lifetimes because for covering this range we need 10 to 15m translation stage, which is not practical. TCSPC is the most common technique for fluorescence lifetime measurements, and it allows us to measures fluorescence decay time from 10ps to a few hundred nanoseconds. It is an elegant technique that uses electronic and a trick to circumvent the inherent core time resolution associated with electronics.
TCSPC technique is based on the detection of single photons of a periodic light signal and the measurement of their arrival times with respect to the excitation pulses. A pulsed laser with a high repetition rate is used to excites the sample. If single-photon events are detected, their time of arrival is correlated to the laser pulse. Then the time difference between the excitation and emission events are measured by the electronics. The repetition rate of the pulsed laser is much higher than the photon detection rate, and only a single photon per signal period needs to be considered. However, measuring from a single excitation-emission cycle will not give accurate results. Therefore, this process needs to be repeated many times to build up a photon distribution over time. To achieve this, the sample is going to be excited periodically, and the data will be collected over multiple excitation-emission cycles to develop the time versus count histogram.
Methodology: Setting up a TCSPC system
The main components of a TCSPC system are a pulse laser, timing device, single photon detector and an optional monochromator. The timing device is connected and synchronized with detector and laser units. Detector can be coupled with a monochromator for the selection of the emission wavelength. The counting electronics process the signal from the detector and laser.
The main components for signal processing in timing device are Constant Fraction Discriminators (CFD), the Time-to-Amplitude Converter (TAC), Analogue to Digital Converter (ADC) and digital memory.
The sample is excited by a with a high repetition rate pulsed laser source, and emitted photons are detected with the highly sensitive fast photodetector. If a single photon is met, the following process will take place in TCSPC timing device: the electronic signal generated in the detector will be directed into CFD, which its job is to initiates a voltage ramp on the TAC. TAC's capacitor will be charged for the time duration between the arrival of a signal at the start and appearance of a signal at stop. Upon the arrival of a signal at the start module, it starts getting charged until another signal appears at the stop module. The signal at stop comes from the pulse laser, which sends the synchronous trigger. The synchronous trigger has the same repetition as the laser itself. TAC linearly ramps a voltage across capacitor once it sees the start signal and it only stops ramping voltage after a photon from the sample is detected. After appearing signal at a stop, it will be discharged across resistance, and the voltage across the resistance is measured. The measured voltage is proportional to the charge has accumulated on the capacitor. In other words, the final voltage after the photon is being detected is directly proportional to the time at which photon arrived.
We say the charges have accumulated on the capacitor is proportional to the time of charging. So, we can write:
Vα Δt, where Δt= time(start) – time(stop) where V is voltage across the resistance.
The analogue to digital converter (ADC) converts that voltage into time. We keep recording Δt and keep track of how many times a particular Δt occurs, and then we plot the number of occurrences versus Δt, which is a histogram. The signal from the ACT goes into the computer with multichannel analyser (MCA) which is then displayed on a histogram. ADC sends the data to memory to bin the value. The whole convoluted process happens during the dead time; this is the time system responding to that photon.
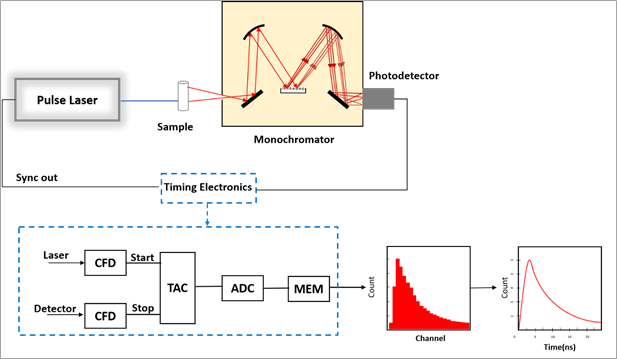
Figure 2: Schematic of the TCSPC setup. The sample is excited by a high repetition rate pulsed laser source. Emitted photons are detected with the highly sensitive fast photodetector, and the timing electronics measure the time with respect to the excitation pulse. By repeating this process and counting many events, TCSPC builds up the distribution of the photons overtime after the excitation pulse. A histogram is collected in a block of memory, where one memory cell holds the photon counts for each corresponding time bin/channel. By joining tips of the histogram, we can get the same curve as excited state population, the decay of fluorescence intensity. Each data point corresponds to the actual histogram of collected photons that comes at a particular time point—conted photons place with bin. The histogram typically shows an exponential decrease in counts as a function. The lifetime is extracted by fitting the data to a single-exponential or multi-exponential function.
From the times of the individual photons, TCSPC builds up the distribution of the photons over the time after the excitation pulse. Every photon seen by the detector contributes to the build-up of the photon distribution. This process is repeated until one channel reaches a pre-set number of counts, usually 10,000. The stop count is kept this large to increase the accuracy, due to the statistical nature of the technique. The basic concept of this technique is that the histogram has the same shape as the excited states decay and fluorescence decay.
Editor’s Notebook
Components for consideration based on application
Light Source
TCSPC is a statistical method requiring a high repetitive pulsed laser to accumulate a sufficient number of photon events for a required statistical data precision. The pulse duration should be in the range of femtoseconds to about a few nanoseconds, and the pulse repition rate should usually be between 1 - 100 MHz. Depends on the lifetime of your sample, different excitation source can be applied. From low-cost pulse, nano LED to semiconductor laser diodes to Femtosecond laser to supercontinuum lasers. In table 1, you can see the advantages and drabacks of using two different excitation sources.
| Pulse Nano LED Laser | Femtosecond Laser |
Fluorescence Lifetime | Few nanosecond | 1ps or greater lifetime |
Cost | Very cheap | Very expensive, bulky |
Exitation Wavelength | Fixed wavelength | Tunable |
Repetition Rate | Variable rep rate | Fixed |
Table 1: Comparison Fetomsecond laser with Nano LED
Table 1. Comparison Femtosecond laser with Nano LED
Detectors
A single-photon detector is employed to record the fluorescence emission. In general, it is tough to detect a single photon or electron due to minimal voltage change they make, so it requires signal amplification. For the single-photon detection system, the following options are available:
-Photomultiplier tube (PMT): although it is inexpensive, it is not suitable for fast detection.
-Microchannel plate (MCP): is a family of PMT with higher resolution, sensitivity and quicker response
-Avalanche photodiode detector (APD): is a semiconductor version of PMT suitable for detection of speedy decay (subject to excitation source is of the same order of magnitude) However, it is costly.
-Streak camera: it can get both spectrum and decay information across the whole range in which it decays, However, these are specialised devices that are not trivial to use, and their usage is principally applied to weakly emitting ultrafast decays. Compared to other detectors, it has higher resolution, fast collection, but it is costly; it also requires a femtosecond laser for high detection.
Suggested Equipment
Laser: NIR Picosecond Lasers (QLD-301)
SIMTRUM offers compact and affordable picosecond laser system suitable for TCSPC. The QLD-301 which is a direct-pulsed diode laser system featuring adjustable pulse width, low time jitter, high extinction ratio and high repetition frequency. Laser pulses can be triggered internally or externally with built in precision delay control. A sync output and a programmable clock output allow to trigger other components such as a TCSPC or testing electronics for single photon system. For more detail’s specification refer to product page.
Timing Device: IDQ Time controller IDQ (id900) - Specifications
We recommend the ID900-TCSPC (time-correlated single-photon counting), which offers time-tagging, counting and histograming continuously on 4 input channels, ideal for all applications requiring time resolved and picosecond event tagging. The ID900 Time Controller is a comprehensive, affordable, versatile and scalable series of instruments. The ID900 Series hardware platform consists of 4 input and 4 output signals that are interconnected internally via a fast FPGA circuit, which is easily programmable via a dedicated user-friendly interface. This unique architecture allows the user to configure customized logical operations between input signals and generate pulsed signals according to programmed rules and send them back to the experimental setup in real time via the available output ports. For more detail’s specification refer to product page.
Detector: Single photon detector IDQ’s ID120 series - Specifications
We recommend IDQ’s ID120 series, which consists of compact and affordable single-photon detector modules, based on reliable silicon avalanche photodiodes that are sensitive in the visible spectral range. These detectors come as free-space module, passive quenching, maximal efficiency value around 800nm. Key advantages of the ID120 modules are high quantum efficiency up to 80%, a large active area and adjustable settings. For more detail’s specification refer to product page.
| 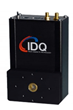 |
We refer you to the webinar from our Partner ID Quantique (top of the page). In this webinar, you will become familiar with Enhancing quantum communication and computing, fluorescence measurements, LIDAR and IC inspection with single-photon detection.
Technical Tips
Linearly polarized pulse laser
If you do not need much time resolution, you can use laser diode, which is the comparatively cheap and easy solution for the light source. However, it is essential to work with an linearly polarized pulse laser. If you are using a diode laser, you need to use a polarizer in the path of the light before it goes into the sample. For femtosecond laser, on the the other hand, the output of the laser is already linearly polarized, and the polarizer is no longer required.
Accurate detection
The detector and electronics have dead-time in which after the first photon detection, they cannot respond. Presence of too many incoming photons causes false detection. Thus, it is imperative to keep the incoming photons very low and allow the system to process the first photon. If too many incoming photons appeared at the detector, they are not going to be counted because the system is too busy to process the initial photon during the dead time. If two photons come before the end of the dead time, the initial photon is detected and the second photon is missed. In other words, the system starts counting the photon that comes first, and cuts off the photon that comes later. If we have too many incoming photons from the sample, this skews the histogram of the photon to earlier time points and changes the shape of the decay. How to avoid?
The webinar from our Partner ID Quantique (top of page). In this webinar, you will become familiar with Enhancing quantum communication and computing, fluorescence measurements, LIDAR and IC inspection with single-photon detection.
SIMTRUM is active in the Photonics field and welcomes any emails for discussions, questions or implementation. We are based in Singapore (+8GMT) and available via Phone or Email. We are especially interested in customised solutions not commercially available.
If you are interested in photon correlation using state of the art equipment for precision, speed and performance, we recommend reading application note by our partner ID Quantique.
Consultation
With over a combined 12 years of experience in the field, Simtrum's R&D team has developed tens of spectroscopic setups with unique capabilities that are not available in commercial instruments. Our project team is focused on practical and elegant solutions. Please contact us if you need any enquiries about time-correlated single-photon counting and setting up experiments.
SIMTRUM Developed TCSPC System for SPAD , APD Testing Solution,
for details working principle and setup please click here